7 Common Types of Audio Background Noise and Their Scientific Origins in Voice Recordings
7 Common Types of Audio Background Noise and Their Scientific Origins in Voice Recordings - White Noise Background Static The Physical Nature of Random Air Molecule Movement
The essence of white noise lies in the erratic dance of air molecules. Their random movements create a consistent sound that encompasses a vast spectrum of frequencies, ranging from the lowest humanly audible (20 Hz) to the highest (20,000 Hz). This constant acoustic presence permeates our daily existence, noticeable in the gentle hum of household appliances or the familiar static on a radio. What distinguishes white noise from other audio disturbances is its capacity to effectively cover up or mask other sounds. This property can be particularly helpful in situations where a person needs to focus or concentrate, whether it's studying or working in a noisy environment. The perceived calming effect of white noise is often attributed to its ability to reduce distractions, potentially promoting relaxation and better focus. The interplay between this physically generated sound and its impact on the human psyche underlines the intricate relationship between background audio and the quality of voice recordings, demonstrating that a deeper understanding of noise is important when it comes to clarity in audio capture.
White noise, with its uniform distribution across the audible frequency spectrum, bears a striking resemblance to the seemingly random dance of air molecules. This molecular jiggle, governed by the fundamental laws of thermodynamics, leads to pressure variations that manifest as sound waves. These sound waves, in turn, can converge to create the perception of a consistent, uniform auditory backdrop – the very essence of white noise.
The human ear is acutely sensitive to changes in sound pressure, making white noise remarkably effective at masking distracting sounds from the environment. This characteristic is why white noise proves beneficial for both improving concentration and promoting restful sleep. In contrast to "colored" noise variants like pink or brown noise, which emphasize specific frequency ranges, white noise sustains a consistent power density across the audible spectrum. This flat power spectrum makes it a valuable tool for audio therapies and for managing conditions like tinnitus.
The genesis of white noise lies in the inherent chaos of natural systems. This showcases the intriguing connections between sound, the principles of thermodynamics, and the statistical behaviors of gas particles. When examined on a microscopic scale, white noise can be visualized as a collection of sine waves. The resultant "superposition" of these waves generates a flat spectral density, a direct echo of the random thermal motion underpinning the phenomenon.
The term "static" often used to describe white noise is, in a way, fitting. It reflects the non-periodic, erratic noise arising from the thermal movement of gas particles, encapsulating the innate randomness inherent in these systems. This consistency in sound is harnessed in a wide array of products, from sleep aids to noise-cancelling headphones, to effectively mask unwanted environmental noises.
Research suggests that sustained exposure to white noise can have a varied impact on cognitive function. For some, it can be an effective tool for enhancing concentration, but for others, depending on intensity and context, it might become a source of distraction. The influence of white noise isn't confined to human perception; it extends to the realm of animal behavior as well. Many species rely on ambient noise to navigate their surroundings and communicate, underlining the broad prevalence of this acoustic phenomenon in the natural world.
7 Common Types of Audio Background Noise and Their Scientific Origins in Voice Recordings - Traffic and Vehicle Sound Waves Low Frequency Urban Sound Patterns
Traffic and vehicle sounds generate a persistent low-frequency hum in urban areas, a significant contributor to the acoustic environment that shapes the experiences of residents. These low-frequency sounds, generally below 200 Hz, have a tendency to travel further than higher frequencies due to less energy loss during propagation. They can create a subtle, yet persistent, background noise in cities. This constant exposure can become bothersome, impacting the overall quality of life for urban dwellers. While often overlooked amidst the overall noise of a city, these low-frequency sound waves play a considerable role in the urban auditory experience.
The characteristics of traffic noise are diverse and can vary considerably based on the types of roads, the vehicles traveling on them, and surrounding environmental features. This leads to a complex and unique soundscape in every urban environment. As cities develop and transportation systems evolve, it's becoming increasingly important to manage and understand how these low-frequency sound patterns influence the urban acoustic environment. Recognizing the impact of low-frequency traffic noise and its origins is crucial for future urban planning and development, as these spaces continuously reshape the soundscape of our cities. The nature of urban noise and the growing understanding of how humans perceive sounds emphasizes the need for thoughtful urban development when it comes to designing acoustic environments that are more beneficial to resident well-being.
Traffic and vehicle sound waves generate low-frequency urban sound patterns, typically below 200 Hz, that can penetrate buildings more effectively than higher frequencies. This makes them particularly intrusive in residential areas, potentially leading to sleep problems and heightened stress due to chronic exposure.
The Doppler effect plays a major role in how we perceive vehicle sounds. As a vehicle approaches, the frequency of its sound increases, and as it passes, the frequency decreases. This principle gives us insights into traffic flow and can be used to predict noise levels in different urban locations. Research has shown that the characteristic "traffic hum" often observed in urban environments tends to reside within the 50-100 Hz range, a crucial factor for acoustic modeling in urban planning to mitigate noise pollution.
The materials used in urban environments, like asphalt and concrete, affect the way vehicle sounds propagate. Rough surfaces tend to scatter sound more than smooth ones, producing complex soundscapes that vary considerably between cities. The noise levels of these low-frequency sounds fluctuate depending on the time of day, with traffic volume naturally influencing the intensity. It's fascinating that nocturnal sound patterns differ significantly, as a reduced number of vehicles lead to a quieter environment.
Beyond human experience, it's important to remember that low-frequency urban noise can negatively impact wildlife. Animals with sensitive hearing, in particular, can face disruptions to communication and mating behaviors. This reminds us of the often-overlooked ecological impact of our human-generated soundscapes.
Urban traffic noise can create "sound masking," where lower frequencies obscure those at higher frequencies. While this is often a design feature to help create environments better suited for focus, if unchecked, it can contribute to negative auditory experiences. However, the impact of sounds isn't solely defined by vehicles. Buildings and other structures play a role, either amplifying or reducing certain frequencies based on their design and materials.
Heavy vehicles, especially large trucks, have a significant contribution to the overall low-frequency noise in urban environments. Their sound levels can exceed those of smaller vehicles due to their weight and speed. This understanding informs urban development guidelines for noise mitigation. As vehicle design shifts, particularly with the growing adoption of electric vehicles, we can anticipate a noticeable change in the urban soundscape. With a likely reduction in low-frequency noise emissions, future cities may face a notable shift in their acoustic environment, potentially prompting adjustments to noise regulations and urban planning practices.
7 Common Types of Audio Background Noise and Their Scientific Origins in Voice Recordings - HVAC System Drone The Physics of Air Circulation Mechanics
HVAC systems, essential for maintaining comfortable indoor environments, are intimately linked to the mechanics of air circulation and, consequently, the acoustic landscape within buildings. One common sonic byproduct of these systems is the "HVAC system drone," a persistent, low-frequency hum that often emerges due to the physics of airflow and the operation of mechanical components like fans and blowers. This drone can have a noticeable impact on the overall auditory experience, and its presence underscores the significance of evaluating and regulating sound levels within buildings to meet recommended standards that prevent excessive noise disturbances in areas where people spend time. Complicating matters further, various malfunctions, such as obstructions in ventilation paths or the presence of trapped air, can introduce more irregular sounds, leading to an even more diverse acoustic environment. The ability to understand and diagnose the origins of these noises, which may manifest as a drone or something more erratic, is important not just for equipment maintenance but also for improving the general audio quality in spaces. By recognizing the links between HVAC system operation and the acoustic environment, we can better understand how background noise influences the clarity of recordings, particularly when capturing human speech.
HVAC systems, whether single-zone or multi-zone, rely on the placement of heating and cooling coils within central air handling units to regulate indoor temperatures. Understanding how air moves within these systems is crucial for optimizing their performance and minimizing any undesirable noise they generate.
One of the fascinating aspects of HVAC systems is the phenomenon of thermal stratification. In larger spaces, air temperature isn't uniform. Warmer air tends to rise, while cooler air sinks. This creates distinct temperature layers that can impact efficiency if not considered during system design. The use of drones equipped with sensors to detect these temperature variations can help optimize heating and cooling, potentially leading to significant energy savings.
The movement of air, or air circulation, is also central to humidity regulation. The physics involved in how air moves through ducts, vents, and rooms plays a key role in regulating moisture content. Advanced HVAC drones with the ability to assess humidity levels at varying heights and locations offer the opportunity for more precise humidity control. This can improve both occupant comfort and protect sensitive materials that are vulnerable to moisture damage.
Airflow patterns themselves can be quite complex. The presence of furniture, structural elements, and even the shape of the space can influence the way air circulates. Phenomena like the Coanda effect, where fluids tend to follow curved surfaces, can guide the design of HVAC systems. Optimizing the placement of vents can improve air distribution and system performance by better accounting for these complex flow dynamics.
Modern HVAC systems often employ adaptive controls based on real-time data. Drones fitted with specialized sensors for airflow can supply data to these control systems. This continuous monitoring can allow for dynamic adjustments to air mixing and distribution, potentially resulting in considerable energy savings.
However, the very forces that enable efficient air circulation can also cause unexpected side effects, particularly when it comes to sound. The variations in air pressure created by HVAC systems can lead to unusual sound propagation effects. Understanding this interplay between air movement and sound is valuable for engineers, helping them mitigate unwanted echoes and background noise. In environments where audio clarity is paramount, such as recording studios, attenuating this HVAC-generated noise becomes a crucial design element.
The shape of the ductwork itself plays a role in the sound profile of an HVAC system. Complex bends or the use of materials with rough surfaces can amplify noise levels. This is especially concerning in areas meant to be quiet, like residences or libraries. Careful selection of duct materials and optimization of duct routing are important design considerations to limit unwanted sound from the HVAC system.
Drones have revealed that buildings can have areas with localized climate differences, so called 'microclimates.' These areas develop due to varying temperatures and airflow. By mapping these microclimates with drone-based sensor data, we can design HVAC systems to be more specific in their control, optimizing comfort and efficiency on a smaller scale.
Understanding how air moves involves understanding the physics of velocity and turbulence. Turbulence, the erratic and chaotic component of fluid motion, can lead to energy losses in HVAC systems. Drones capable of measuring turbulence and airflow velocity can give engineers more insight into where improvements to system design can reduce wasted energy.
The concept of acoustic impedance is another facet of air circulation that matters for sound management. Acoustic impedance is a measure of how much a material resists sound propagation. Leveraging an understanding of this principle helps HVAC designers limit the possibility of generating undesirable noise, especially in acoustically sensitive locations.
Finally, the use of drones for data collection is changing the way we think about HVAC system maintenance. Predictive maintenance—identifying issues before they become a problem—becomes feasible with the information provided by drones. This shift from reactive to proactive maintenance allows for greater operational reliability and potentially reduces the overall need for disruptive maintenance repairs, maximizing system longevity and minimizing noise disturbances.
The increased adoption of drones within the HVAC field is expected to continue as they offer advantages in energy audits, safety inspections, and operational efficiency. Their role in characterizing and controlling airflow within HVAC systems allows for better design, refined system operation, and a reduction in unwanted acoustic noise. This demonstrates that the interaction between airflow, sound, and drone technology is an important area of ongoing research and development in the HVAC field.
7 Common Types of Audio Background Noise and Their Scientific Origins in Voice Recordings - Echo and Room Reverberation Sound Wave Reflection Principles
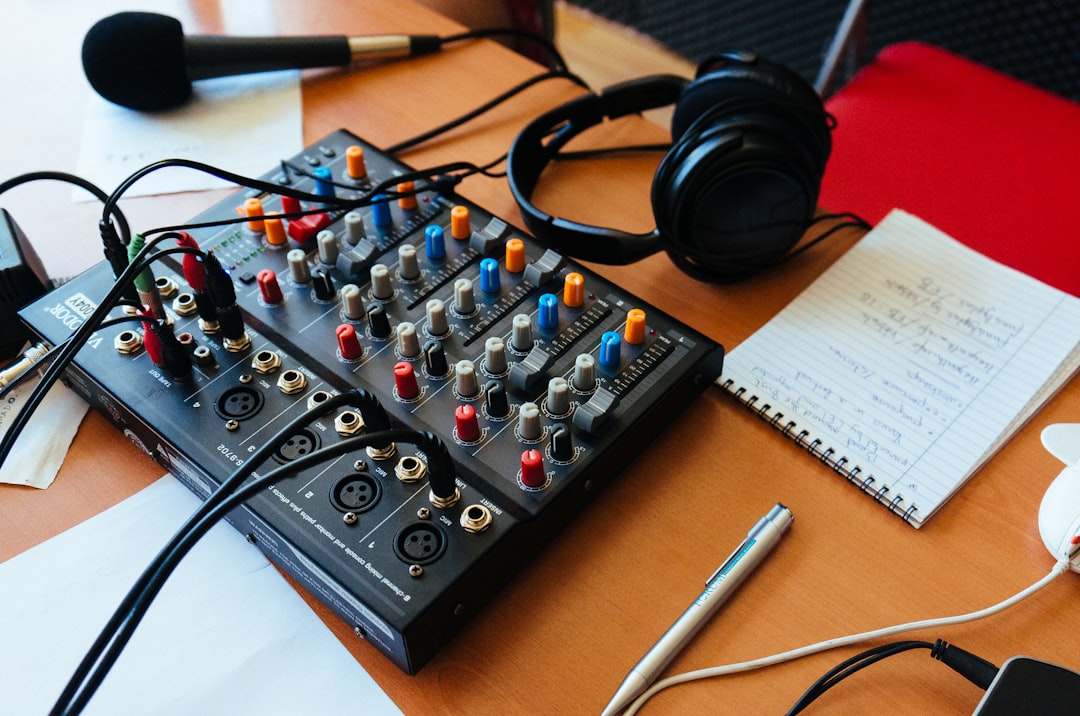
Echo and room reverberation are fundamental aspects of how sound waves interact with their surroundings, significantly impacting the audio quality of voice recordings. Echo is a distinct phenomenon where sound waves bounce off surfaces and return to the listener, following the basic principle that the angle at which the sound hits a surface equals the angle at which it bounces off. Reverberation, on the other hand, is a more complex blend of overlapping sound reflections. Instead of distinct echoes, it generates a continuous, lingering sound, influencing how we perceive the overall audio landscape. The nature and extent of both echo and reverberation are shaped by a variety of factors. These include the dimensions of the room, the materials used on its surfaces, and the distance between the sound source (like a speaker) and the listener. These elements influence how effectively sound waves are reflected and absorbed, thereby shaping how clear and understandable speech and other sounds become. This intricate interplay of sound wave reflections and absorption plays a major role in the acoustic characteristics of a space. Understanding these principles is crucial for managing sound environments, especially when voice recordings are concerned. Background noises can be significantly affected by room reverberation and the characteristics of echoes, which can ultimately either enhance or degrade the perceived quality of the recordings.
Echoes and room reverberation are fascinating consequences of sound wave reflections. The basic principle is straightforward: sound waves bounce off surfaces, following the law of reflection where the angle of incidence equals the angle of reflection. However, the experience of hearing a distinct echo is different from experiencing reverberation. While an echo is a clear, delayed repetition of a sound, reverberation is a blend of overlapping reflections that we perceive as a continuous, lingering sound.
The characteristics of a room heavily influence how we experience both echoes and reverberation. Factors like the room's size, the materials of its surfaces, and the distance between the sound source and listener all play a role. For example, a larger space naturally takes longer for sound to reflect, leading to longer reverberation times. This is why concert halls are designed with specific reverberation times to enhance the musical experience. Curiously, the time delay in the return of reflected sound waves is critical to our perception. If it's less than about 0.1 seconds, our ears blend the reflections into a reverberant experience rather than hearing a distinct echo.
The materials of a room also play a significant role in the reverberation time. Materials with different 'absorption coefficients' will absorb sound to varying degrees. Think of a soft, sound-absorbing carpet compared to a hard, reflective wall. The soft carpet will noticeably dampen echoes and reverberation by soaking up the sound wave energy.
Another fascinating concept is the 'critical distance,' a point where direct sound and the combined reflected sounds become equally loud. This is a crucial factor in voice recording situations. Beyond the critical distance, reflected sound dominates, and the original source of the sound may become less important to the overall audio experience.
The point where the sound first reflects off a surface – the first reflection point – also impacts how sound is perceived. Understanding and potentially controlling this point is crucial in audio engineering where reducing undesired reflections and improving clarity are paramount. Early reflections, while a vital part of our perception of space and depth, can have adverse impacts if they're too delayed. This can significantly impact the clarity of speech, as we can hear multiple versions of a sound arriving at slightly different times. When these reflections overlap, the result can be a phenomenon called "phantom imaging," where we perceive sound as originating from somewhere other than its true source.
Interestingly, the whole study of sound reflection and reverberation in environments has led to specialized environments called reverberation chambers, specifically designed to test and understand the complexities of sound wave interaction. Within these chambers, researchers can investigate how reflections create standing waves (the reinforcing of reflected waves). These standing waves, often associated with room modes, produce resonant frequencies in specific locations, leading to potentially uneven sound distribution, impacting the overall quality of sound in a space.
In conclusion, the interplay of echo and reverberation with room characteristics—size, material absorption, and geometry—demonstrates the intricate relationship between sound waves and the environment. This understanding is central to areas such as acoustics, audio engineering, and architecture, particularly in instances where clear communication and high-quality recordings are crucial, as is the case in voice recording applications.
7 Common Types of Audio Background Noise and Their Scientific Origins in Voice Recordings - Electronic Device Interference Electromagnetic Field Disruptions
**Electronic Device Interference: Electromagnetic Field Disruptions**
Electronic devices, a ubiquitous part of modern life, can introduce unwanted electromagnetic interference (EMI) into audio recordings. This interference stems from a variety of sources, ranging from everyday appliances and communication networks to more powerful events like lightning strikes. One common type, known as common mode interference, occurs when stray electromagnetic energy impacts the wires carrying audio signals, producing noise and signal distortion. Furthermore, transient interference, or impulse noise, generated by electrical power fluctuations or rapid switching of devices, can also manifest as abrupt, short-lived disruptions in the recording. These interference patterns, whether persistent or transient, can negatively affect the quality and clarity of audio recordings. Successfully recognizing and isolating these interference sources is essential for improving audio quality and implementing effective methods to reduce unwanted noise in voice recordings.
Electronic devices, from Wi-Fi routers to computers, generate electromagnetic fields (EMFs). These EMFs can interfere with audio equipment, introducing unwanted noise into recordings. This interference can manifest as distortion, static, or other unexpected sounds, highlighting the impact of these invisible fields on the quality of audio captured by microphones and recording devices.
Many audio components, including microphones and recording interfaces, operate within frequency ranges that overlap with common sources of EMI, such as power lines and electric motors. This overlap can lead to distinct, unwanted sounds in recordings. Consequently, engineers often employ shielding and filtering techniques to reduce the impact of EMI.
The strength of the EMI signal tends to decrease as the distance from the source increases. This means that placing a recording device close to a strong source of EMI, like a computer or fluorescent lighting, will likely result in greater interference compared to a device positioned further away. Understanding the spatial relationship between the recording equipment and potential EMI sources is crucial for achieving clean audio.
Digital audio systems, in contrast to analog ones, often experience EMI differently. It can manifest as digital artifacts, such as clicks and pops, instead of the continuous noise observed in analog systems. This difference reveals the varying susceptibility of different audio technologies to the effects of electromagnetic disturbances.
When multiple devices operate near each other, EMFs can cause "crosstalk." This phenomenon results in signals from one device leaking into another, potentially interfering with the desired audio. This is a particular concern for multi-channel recordings, where unintended sounds can mask the intended audio.
Shielding materials play a vital role in mitigating EMI. Some materials, like mu-metal, which has high magnetic permeability, are particularly effective at reducing EMI. However, other common materials like plastic or standard steel are less effective in this regard.
Electromagnetic Compatibility (EMC) standards have become increasingly important in audio equipment design. These standards aim to ensure that devices both minimize their own EMF emissions and function properly when exposed to EMI from other devices. It creates a layer of complexity in the design of audio equipment.
Even wireless phones not actively transmitting signals can still create EMI. This can be a problem, especially in urban areas, where mobile phones are plentiful. The standby emissions of these devices can interfere with recordings, highlighting the need for careful planning of recording environments to minimize the effects of unintended EMFs.
Temperature plays a fascinating, yet complex role. Changes in temperature can affect the electrical properties of materials within audio equipment, potentially altering their sensitivity to EMI. As a device warms or cools, materials within it expand or contract, influencing its susceptibility to EMI in ways that aren't always predictable.
The human body acts as an antenna to some extent, capturing and potentially re-emitting EMI. This can introduce noise into recordings, particularly when people are close to sensitive microphones. Understanding the spatial dynamics and impact of the human body within a recording environment becomes crucial for optimizing recording setups.
This exploration of electronic device interference illustrates how complex and varied the sources of unwanted noise in audio recordings can be. It's a constant challenge for engineers and researchers working on better ways to isolate audio and manage interference to capture clean, undistorted audio signals.
7 Common Types of Audio Background Noise and Their Scientific Origins in Voice Recordings - Microphone Self Noise Internal Circuit Sound Generation
**Microphone Self Noise Internal Circuit Sound Generation**
Every microphone, even in complete silence, generates a faint internal sound known as self-noise. This noise originates from the random movement of electrons within the microphone's circuitry. You might perceive it as a subtle hiss or a rushing sound. The level of this self-noise can significantly impact the clarity of audio recordings, particularly when trying to capture quiet sounds.
The quality of a microphone is partly judged by how low its self-noise is. High-end microphones typically produce a self-noise that's barely audible, often between 3 and 10 decibels (dBA). However, many other microphones can have significantly higher self-noise levels, ranging from 10 to 20 dBA. Environmental factors, like temperature and electrical resistance (impedance), can also play a role in determining the microphone's self-noise.
For audio professionals focused on capturing clear voice recordings, understanding self-noise is essential. The combination of a microphone's self-noise and its sensitivity determines the quietest sound it can effectively capture without its own internal noise becoming dominant. This interplay between internal noise and sensitivity is crucial when selecting a microphone for any application where high fidelity audio is needed. This means that, if you are trying to capture very soft sounds, the microphone's own internal noise could be a major obstacle to getting a good, clear recording.
Microphone self-noise is essentially the inherent sound a microphone produces when there's no external sound to pick up. It's a consequence of the random jiggling of electrons within the microphone's internal circuits, a phenomenon driven by thermal energy. This noise often manifests as a faint hiss or a rushing sound, especially noticeable in sensitive microphones. We use a metric called Equivalent Noise Level (ENL) measured in decibels to quantify this intrinsic noise – it's essentially the noise output in absolute silence.
The type of transistors employed in a microphone's circuit has a huge impact on self-noise. While field-effect transistors (FETs) are often chosen to minimize noise, they still generate some, which becomes more noticeable when dealing with soft sounds. This becomes a concern especially with condenser microphones which require phantom power to operate. The quality and stability of that phantom power can itself impact the overall noise, with fluctuations leading to more noise. Different types of mics have different noise profiles. For example, dynamic mics, with their simpler designs, tend to be quieter than condenser mics. Ribbon microphones, however, are particularly susceptible to electromagnetic interference, which can worsen their inherent noise.
The self-noise floor, the lowest level of noise a recording system has, is significantly influenced by a microphone's self-noise. A microphone with a low noise floor allows capturing fainter sounds without being drowned out by the mic's own electronic fuzz. It's also interesting to note that we tend to be more sensitive to microphone noise in recordings of speech than we are in recordings of music. It seems our ears are primed for clarity when it comes to speech, and any extra noise becomes more noticeable.
Temperature is another critical factor, as higher temperatures lead to more electron jiggling, which in turn, amplifies self-noise. This means a microphone's performance might vary in different environments, making it important to consider temperature control in recording setups. The age of the microphone matters too. Components can degrade over time, particularly in condenser microphones where aging capacitors can contribute to increased noise. Researchers are continually exploring ways to improve microphones using innovative materials like specialized low-noise resistors and circuit boards to reduce these internal noise sources.
Measuring microphone self-noise is a crucial part of design and quality control. It involves highly controlled environments to eliminate outside noise so that we can truly measure a mic's inherent noise floor. These tests provide vital data for evaluating how well a microphone is designed and how it will perform in different conditions. Ultimately, a deeper understanding of microphone self-noise is critical for audio professionals, especially in applications where high fidelity and capturing soft sounds are important, like in voice recordings or transcription.
7 Common Types of Audio Background Noise and Their Scientific Origins in Voice Recordings - Wind Distortion Aerodynamic Pressure Fluctuations
Wind distortion, specifically the aerodynamic pressure fluctuations caused by wind, can significantly interfere with audio recordings, particularly when recording outdoors. These fluctuations are a product of the complex interactions between the movement of air and sound waves. The turbulence generated by wind can obscure the sounds we're trying to capture, resulting in poor signal quality or a low signal-to-noise ratio. This makes it difficult to clearly hear what's being recorded.
Furthermore, the direction and intensity of wind can impact how sound travels, making it harder to consistently evaluate audio quality in real-world settings. This challenge is exacerbated by the unpredictable nature of wind itself. These complexities underscore the need to develop and employ strategies that minimize the impact of wind noise on recordings, allowing us to capture clear and reliable audio in a wide range of environments, especially when dealing with natural environments that can have significant wind variations.
### Surprising Facts About Wind Distortion Aerodynamic Pressure Fluctuations
1. Wind distortion isn't consistent; the pressure changes and resulting sound vary significantly depending on things like the land's shape, plants, and human-made structures. This variability makes it tough to predict what the sound environment will be like in different areas.
2. The pressure changes caused by wind can create sounds across a wide range of frequencies. Surprisingly, these sounds can include low frequencies that travel far and high frequencies that fade out quickly, creating complex sound interactions.
3. When wind speeds approach the speed of sound, it can create strong sonic booms and pressure changes, even if the wind doesn't feel particularly strong. This, influenced by the Mach number, shows how speed and pressure work together to make sound.
4. The way turbulent wind interacts with structures can produce chaotic sound patterns. The complex behavior of airflow around objects leads to nonlinear dynamics that cause unpredictable auditory effects, making it difficult to accurately model and predict sound generation.
5. Buildings and infrastructure can resonate due to wind-caused pressure variations, creating specific acoustic signatures. This resonance can amplify sound levels, highlighting the intricate connection between structures and wind noise.
6. Aerodynamic pressure changes can mask animal vocalizations, particularly in open spaces. This interference can impact mating and social interactions, demonstrating that wind noise has a broader ecological impact beyond human perception.
7. At high altitudes, the combination of thinner air and faster wind speeds results in different acoustic profiles compared to the ground. This means engineers must account for altitude-specific factors in projects involving sound transmission.
8. The random nature of wind flow turbulence can lead to sporadic bursts of sound, complicating sound prediction. This inherent randomness is part of what makes wind-induced noise difficult to manage in recordings.
9. Research in how fluids and structures interact is increasingly incorporating acoustics, focusing on how design changes can minimize unwanted noise. New materials and shapes are being investigated to reduce the acoustic impacts of wind on structures.
10. The low-frequency noise produced by wind turbines is mainly caused by aerodynamic pressure changes created by blade movement. This noise can travel long distances, impacting regulations and turbine placement in residential areas.
More Posts from transcribethis.io: