Mastering the Art of Resonance Detection 7 Practical Techniques for Audio Engineers
Mastering the Art of Resonance Detection 7 Practical Techniques for Audio Engineers - Frequency Sweep Analysis Using Sine Waves
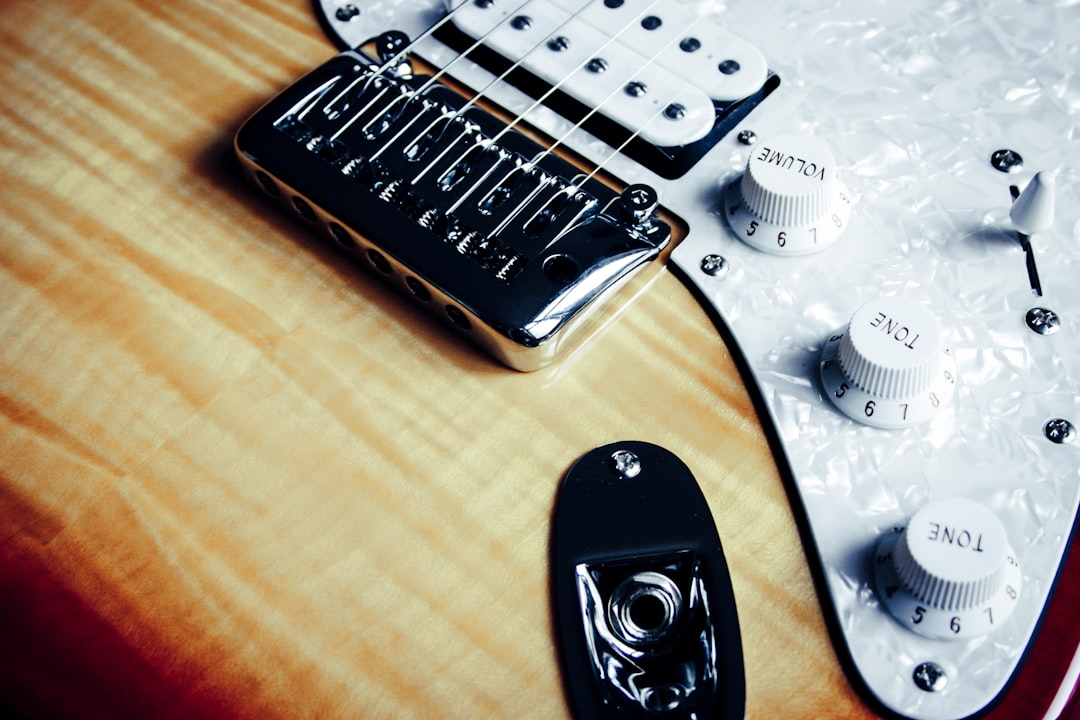
Sine wave sweeps are a powerful tool for audio engineers to identify resonances in a variety of objects. By systematically varying the frequency of a sine wave and observing the response of the object, we can pinpoint the frequencies at which the object vibrates most strongly. These resonant frequencies are crucial to understand as they can influence the sound quality of a product, leading to unwanted noise or even structural damage. The way we perform the sweep, the speed at which we change the frequency, has a significant impact on the accuracy of the results. Slower sweeps allow for more precise measurements and a better understanding of how the object responds to different frequencies. Logarithmic sweeps, which gradually increase the frequency over time, are often used to simulate the type of vibrations that objects encounter in real-world scenarios. This helps to ensure that our analysis accurately reflects how the product will behave in its intended environment.
Sine wave frequency sweep analysis is a common tool in the audio engineer's arsenal. It allows us to delve into the heart of how a system reacts to different frequencies. The beauty of sine waves lies in their purity - they contain a single frequency without any distracting harmonics. This purity allows for focused analysis, enabling us to clearly identify the system's resonant frequencies. These are the frequencies where the system's response is amplified, which can be exploited for desired effects or addressed to avoid unwanted distortions.
By systematically varying the frequency of the sine wave, we essentially trace the system's frequency response curve. This curve reveals the highs and lows of the system's amplification and attenuation of different frequencies. Understanding this curve is vital for refining designs, troubleshooting issues, and optimizing sound reproduction.
Beyond identifying resonances, frequency sweeps offer a peek into the realm of phase cancellation. This phenomenon, where multiple sound waves interact and cancel each other out, highlights the importance of understanding frequency sweep's impact on sound wave propagation in different spaces. This knowledge can be invaluable when designing concert halls, studios, and other acoustically sensitive environments.
The application of frequency sweeps goes beyond analyzing the present. They can also be used to monitor material degradation over time. Slight shifts in resonant frequencies over time can indicate wear and tear, signaling the need for maintenance or replacement.
Finally, frequency sweeps are a powerful tool in investigating acoustic impedance. This measure defines the resistance a medium offers to sound wave propagation. Understanding acoustic impedance is crucial for designing speakers, microphones, and other audio components that efficiently transfer sound energy.
In essence, frequency sweep analysis with sine waves offers a multifaceted approach to understanding sound and its interaction with various structures and materials. It allows us to diagnose problems, explore solutions, and refine our audio design strategies to achieve better sound reproduction and acoustic control.
Mastering the Art of Resonance Detection 7 Practical Techniques for Audio Engineers - Real-Time Spectrum Analyzers for Quick Identification
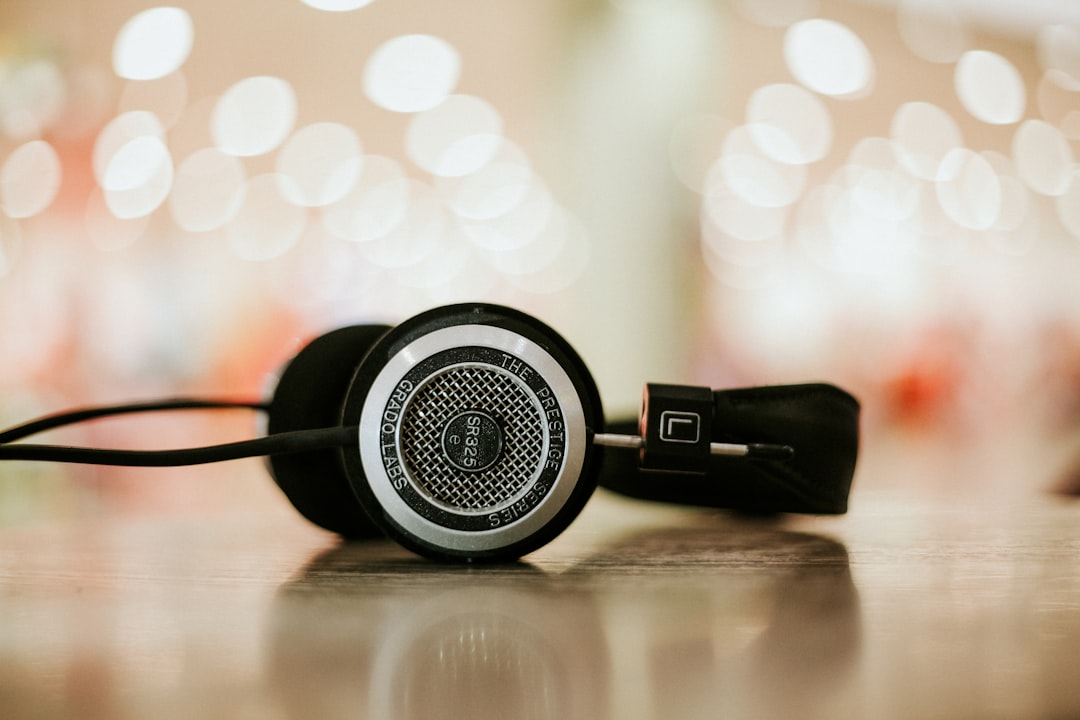
Real-time spectrum analyzers are like x-ray vision for audio engineers, offering a live window into the frequency landscape of their sound. This dynamic view lets them spot problems fast, like frequencies clashing or a mix that's lopsided. With this information, they can make smarter decisions during the production process, whether it's balancing a mix, fixing a muddy sound, or identifying the source of a unwanted rumble.
There are spectrum analyzers for every budget and need. You'll find sophisticated plugins like Voxengo SPAN, which offer a lot of features, and simpler, free options like Blue Cat's FreqAnalyst, which do the job just fine. Ultimately, the best choice depends on what you need and how you prefer to work.
The real magic of these analyzers is their ability to show what's happening in real-time. This helps not only in mixing and mastering, but also in understanding acoustics. By seeing the frequencies involved in a space or instrument, engineers can diagnose issues and make adjustments to achieve better sound. Starting with a neutral, flat frequency response as a base makes these tools even more powerful, enabling precise adjustments that fine-tune the sound.
Real-time spectrum analyzers are invaluable tools for audio engineers seeking to swiftly identify problematic frequencies. Unlike traditional methods that might miss subtle issues lurking beneath the surface, these analyzers reveal the entire audio spectrum simultaneously. Their speed is due to the application of Fast Fourier Transform (FFT) algorithms, which efficiently break down complex waveforms into their constituent frequencies.
However, understanding the influence of resolution bandwidth (RBW) settings is crucial. Narrower RBW settings provide greater detail and can uncover minute variations in frequency response, vital for in-depth analysis.
Modern spectrum analyzers often feature color-coded visualizations, enhancing visual clarity and facilitating quick identification of problematic frequencies. This allows for efficient collaboration and real-time communication amongst team members.
Advanced analyzers incorporate Waterfall displays, enabling time-based analysis of frequency changes. This allows engineers to track transient acoustic events or shifts in resonance over time, providing a more dynamic understanding of the audio environment.
Beyond identifying and adjusting resonant frequencies, real-time analysis plays a crucial role in minimizing feedback during live performances. By swiftly detecting and correcting these frequencies before they create unwanted feedback loops, engineers ensure a smoother and more enjoyable listening experience.
These tools can also be employed to analyze harmonic content. By studying the harmonics of fundamental frequencies, engineers gain insights into how different materials affect sound production, leading to optimized microphone and speaker placement.
High sampling rates further enhance the efficiency of frequency identification. Analyzers sampling at rates exceeding 44.1 kHz capture transient peaks with greater accuracy, crucial for fine-tuning sensitive audio equipment.
Real-time spectrum analyzers can seamlessly integrate with digital audio workstations (DAWs), enabling multifaceted audio analysis and processing. This allows engineers to automate corrective measures based on real-time data feeds, streamlining workflow and enhancing precision.
Furthermore, the development of portable real-time spectrum analyzers with built-in microphones has revolutionized on-site assessments. Engineers can now measure acoustic environments in situ, replacing reliance on static data collected in controlled spaces. This allows for more accurate and realistic assessments of acoustic performance, leading to more effective solutions.
Overall, real-time spectrum analyzers represent a significant advancement in audio engineering, empowering engineers with real-time insight into the frequency landscape of their work. This empowers them to make quicker, more informed decisions and optimize sound production for a superior listening experience.
Mastering the Art of Resonance Detection 7 Practical Techniques for Audio Engineers - Impulse Response Measurement Techniques
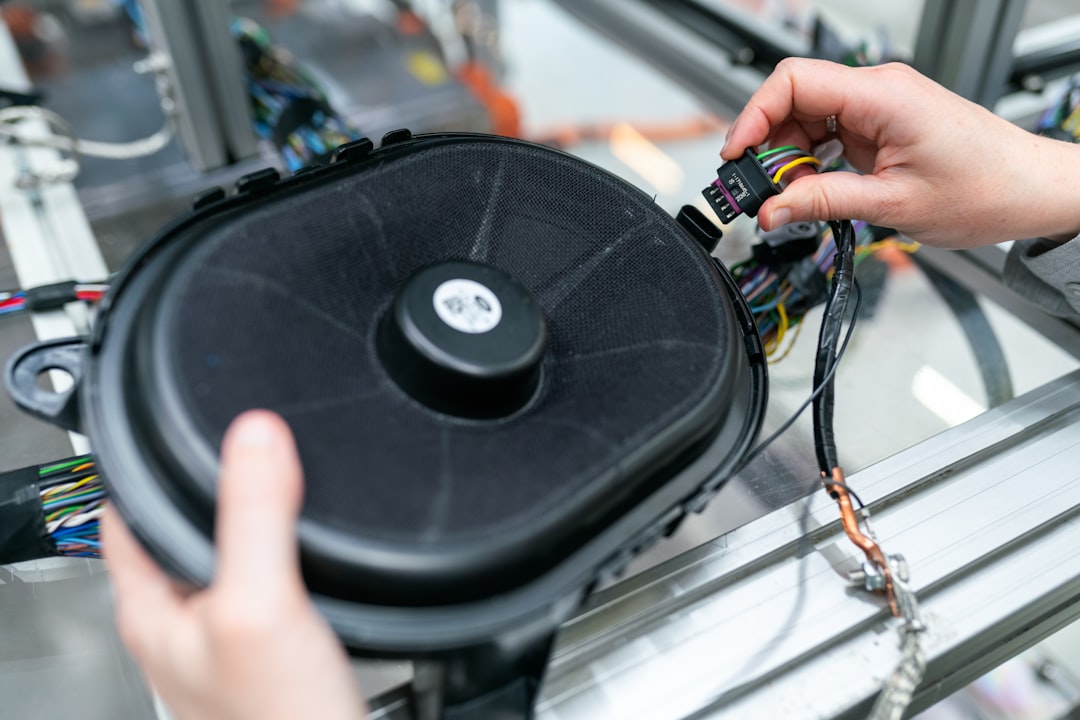
Impulse response measurement is an essential tool for any audio engineer. It provides the vital information needed to understand and improve the acoustics of any space. It allows for the creation of accurate audio simulations and the identification and control of unwanted resonances.
There are several different ways to measure impulse responses, each with its own benefits and drawbacks.
**Maximum Length Sequences (MLS)** utilize pseudo-random signals to capture the full range of frequencies within the space. This technique is quick and accurate but can be challenging to implement properly.
**Exponentially Swept Sines**, as the name implies, uses a sweeping sine wave to analyze frequencies. This approach allows for a wide frequency range to be captured with a single sweep and can be more forgiving than MLS when it comes to setup and accuracy. However, it can miss transient details that can be important for fully understanding the space.
**Time Delay Spectrometry** focuses on the time delays of sounds as they travel through a space. This method can identify specific acoustic characteristics, such as reverberation time and the location of reflecting surfaces. It can be more challenging to interpret the data from this technique, but it can provide useful information about the sound-scattering properties of the room.
Other techniques include **Time Reversal** and **Time-Stretched Pulses**, both of which aim to precisely capture the impulse response for specific purposes. Time Reversal is often used to focus sound energy in a particular location and Time-Stretched Pulses are employed for precise measurement in specific scenarios.
The continued study of impulse responses has helped lead to the development of powerful audio engineering tools, including algorithms for improving audio clarity and optimizing resonance control. There is still a great deal of ongoing research in this field and with each new advancement we become closer to better understanding and manipulating sound.
Impulse response measurements are a vital tool for audio engineers to understand how sound interacts with various systems and environments. They offer a different perspective than traditional frequency sweep analyses, providing a more comprehensive view of a system's behavior, particularly in terms of its response to short, impulsive signals.
These measurements can be categorized into two main approaches: time-domain and frequency-domain. Time-domain methods, like using a short-duration signal, capture the system's response over time, revealing its dynamics and transient behavior. Frequency-domain methods, on the other hand, analyze the response at specific frequencies, offering insights into how the system behaves at different sonic ranges.
Unlike frequency sweeps that focus on steady-state responses, impulse responses can unveil nonlinearities within a system. This means they can expose subtle distortions and how audio equipment behaves under varying loads, something that might be missed by conventional sweep analysis.
The accuracy of impulse response measurements is heavily influenced by the surrounding environment. Reflections from walls, ceilings, and other surfaces can dramatically affect the results. Understanding these reflections is crucial for addressing modes, echoes, and specific resonances that impact sound quality in a space.
Precise measurements demand meticulous attention to the time of flight of sound waves. Environmental factors like air temperature and humidity can affect the travel time of sound, requiring consistent conditions for reliable data.
To accurately capture the fast transients present in impulse responses, advanced measurement techniques often employ extremely high sampling rates, sometimes exceeding 192 kHz. This enables the capture of brief sounds occurring in fractions of a second, essential for high-fidelity audio applications.
The application of impulse response measurements extends beyond analyzing sound. They play a crucial role in digital room correction systems. These systems capture the unique acoustic signature of a space and use impulse responses to adjust playback signals, effectively optimizing the listening environment by mitigating undesirable resonances.
Auralization, the process of creating virtual acoustic environments using impulse response data, enables engineers to simulate how sound interacts within a space before any physical installation. This is particularly valuable in architectural acoustics, allowing for improved design decisions that enhance sound quality.
Impulse responses form the backbone of convolution reverb effects in digital audio processing. By convolving recorded impulse responses of real spaces with audio signals, engineers can digitally recreate the sounds of specific environments, achieving realistic reverberation effects.
The field is evolving with new techniques that combine impulse response measurements with machine learning algorithms. These innovative approaches analyze vast datasets of impulse responses, utilizing AI to predict acoustic behavior in complex environments and optimize audio setups.
While much emphasis is placed on understanding how a system's response affects amplitude, it's important to remember that impulse responses also provide information about a system's phase response. Understanding these phase relationships is crucial for achieving coherent sound reproduction, particularly when combining multiple audio sources, impacting perceived sound quality and imaging.
Mastering the Art of Resonance Detection 7 Practical Techniques for Audio Engineers - Multi-Microphone Array Method for Room Resonance
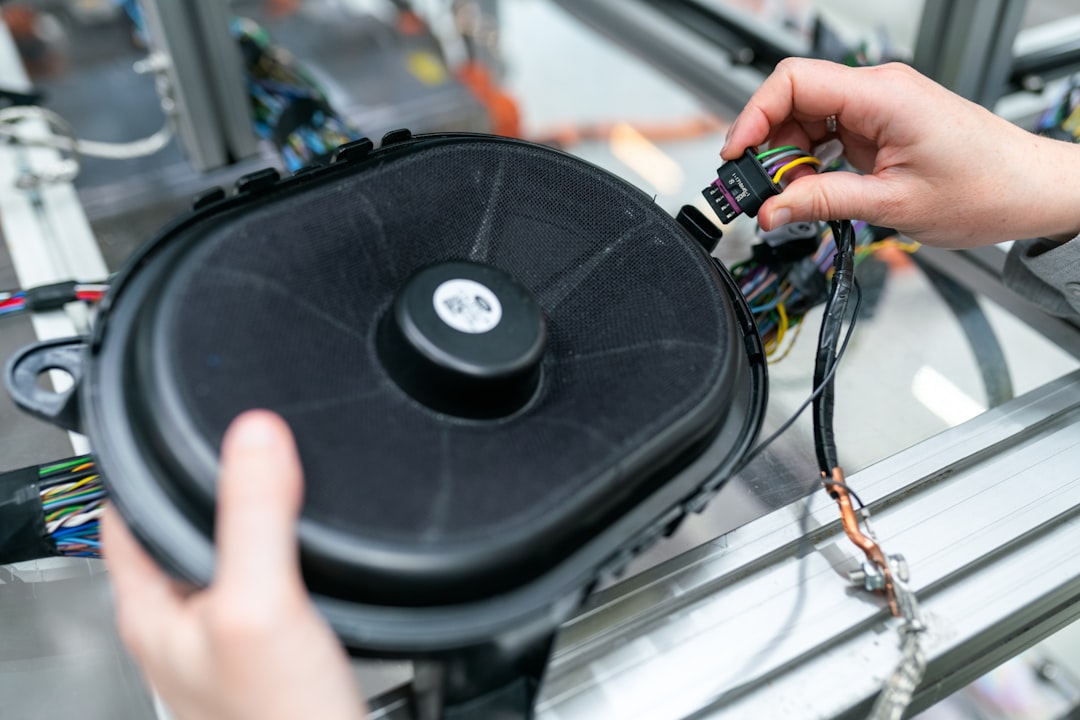
The Multi-Microphone Array Method takes a more advanced approach to detecting room resonances, leading to improved audio signal processing. By using multiple microphones strategically arranged in an array, engineers can gather a more complete spatial understanding of how sound behaves in a room. This helps them better identify room impulse responses, which reveal how sound bounces around in the space. This method also allows engineers to create specific sound capture patterns using uniform circular arrays, optimizing how well the microphones pick up sound. Spherical microphone arrays offer even greater detail, providing a detailed picture of the sound field. In contrast to using just one or two microphones, this multi-microphone approach allows for a deeper understanding of the acoustics in a room, making resonance detection much more accurate.
The Multi-Microphone Array Method delves into the intricate world of spatial acoustics, providing a more nuanced understanding of how sound waves interact within a given space. By employing multiple microphones strategically positioned throughout the environment, engineers can capture intricate sound patterns that a single microphone might miss.
This method reveals nuances in acoustic behavior by exploiting the principles of wave interference and diffraction. The placement and configuration of the microphones, whether in linear, circular, or grid arrangements, can significantly influence the accuracy of room resonance detection. The importance of thoughtful placement underscores the complexity and interconnectedness of these analyses.
A key consideration is phase alignment between the microphones within the array. Any misalignment can introduce phase cancellation, where sound waves from different sources interfere with one another, potentially masking resonant frequencies. This necessitates meticulous calibration of the microphone array, a crucial step in ensuring data integrity.
Beyond simple resonance detection, multi-microphone arrays can implement real-time beamforming techniques. These techniques allow the system to dynamically focus on sounds emanating from specific directions, effectively isolating desired frequencies and minimizing background noise. This proves particularly valuable in live sound environments where clarity is paramount.
An intriguing application of this method is in the realm of binaural audio. By mimicking the way human ears capture sound, multi-microphone arrays can create spatial audio experiences that enhance immersion in virtual environments. This showcases the versatility of this technique beyond its traditional uses in acoustic analysis.
The processing power required to analyze data from multi-microphone arrays is substantial. Advanced algorithms, like adaptive filtering and machine learning, are often employed to efficiently process the collected data. This demand for computational resources highlights the need for sophisticated hardware and software capabilities to ensure accurate real-time analysis.
One unexpected benefit of multi-microphone arrays is their potential in diagnosing structural issues. By assessing how sound waves interact with various surfaces, engineers can glean insights into the integrity and acoustic properties of the structure. This adds a layer of depth to the analysis, revealing information beyond the traditional focus on sound clarity and resonance.
When combined with impulse response measurements, multi-microphone arrays significantly enhance the precision of acoustic simulations. This data allows for the development of highly accurate digital models for acoustic correction setups, leading to more effective and efficient sound optimization in spaces.
The data collected from multi-microphone arrays can also reveal room modes—specific frequencies at which a room naturally resonates. Understanding these modes allows engineers to adjust acoustic treatments, effectively controlling unwanted resonances that might disrupt sound clarity.
Finally, despite its sophistication, the Multi-Microphone Array Method relies on a solid understanding of fundamental acoustic principles. Engineers must adapt their approach based on the specific characteristics of each environment, recognizing that technology alone cannot replace the need for rigorous scientific understanding and hands-on experience in the realm of audio engineering.
Mastering the Art of Resonance Detection 7 Practical Techniques for Audio Engineers - Waterfall Plots to Visualize Decay Times
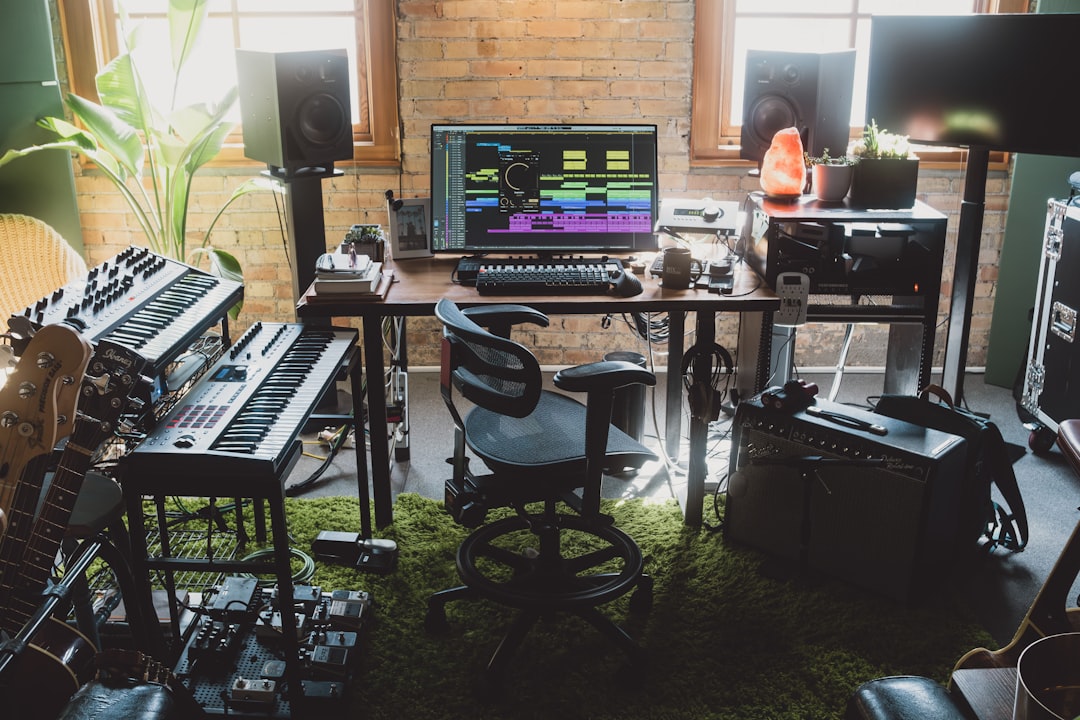
Waterfall plots, also known as Cumulative Spectral Decay (CSD) plots, are essential tools for audio engineers to understand how sound decays over time. They display how sound energy decreases at different frequencies in a room or within a loudspeaker after the initial sound is emitted. Waterfall plots are visual representations of frequency and time, revealing important information like room modes and standing waves that can impact sound quality. The presence of these modes can cause certain frequencies to decay more slowly, potentially leading to muddy or uneven sound. The detailed analysis provided by these plots helps audio engineers optimize speaker placement and room acoustics, ultimately contributing to a better listening experience.
Waterfall plots, also known as Cumulative Spectral Decay (CSD) plots, are a valuable tool for visualizing how sound decays over time. These plots offer insights into how sound behaves in a room after the initial sound emission, revealing important information about room acoustics and speaker performance.
A waterfall plot displays amplitude against frequency on one axis and time on the other. This unique presentation allows audio engineers to easily identify resonant frequencies within a system or space. Each horizontal line in a waterfall plot represents the amplitude of a specific frequency over time, providing a visual representation of how quickly that frequency decays.
Waterfall plots offer several advantages over traditional frequency response graphs:
* **Temporal Resolution**: They can clearly show how decay times change over time and frequency.
* **Non-Linear Behaviors**: They can uncover irregularities in a system's response, like frequencies decaying at different rates, which simpler frequency responses might miss.
* **Room Modes**: These plots can effectively reveal room modes—resonant frequencies where sound energy builds up in a space—leading to better informed acoustic treatment strategies.
* **Phase Relationships**: Waterfall plots can illustrate phase relationships between frequencies as they decay, helping identify cancellation issues or constructive interference that might affect the clarity of a sound.
Modern audio analysis software has made waterfall plots readily available for audio engineers. However, these plots can sometimes be overwhelming. Learning to effectively interpret their complex visual data is essential for accurate analysis.
While the idea of using waterfall plots to visualize decay dates back to the early days of digital signal processing, their continued relevance underscores their importance to sound engineering. Waterfall plots can help engineers:
* **Identify problem areas**: Quickly pinpoint frequencies that have excessive decay times, potentially affecting sound quality.
* **Make informed decisions**: Apply corrective measures to problematic spaces, optimize speaker placement, and refine audio recordings.
* **Improve listener experience**: Contribute to achieving a more natural and enjoyable listening experience by manipulating sound decay times within a given space.
The use of waterfall plots for A/B comparisons is a growing practice. Overlaying plots from different systems or setups can help engineers directly compare decay characteristics and make better informed decisions. Despite the growing use of more sophisticated software, understanding the basics of acoustic theory and principles remains essential for successful audio engineering.
Mastering the Art of Resonance Detection 7 Practical Techniques for Audio Engineers - Pink Noise Excitation for Broadband Detection
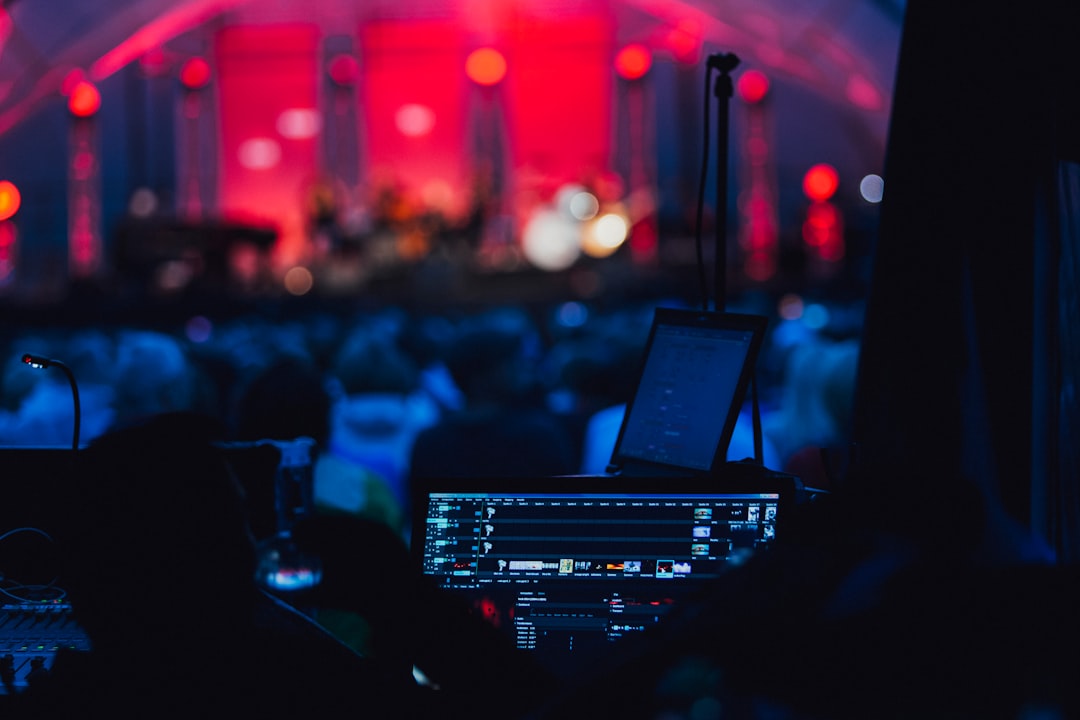
Pink noise offers a valuable approach for detecting a wide range of frequencies, or "broadband" detection. Unlike white noise, which has equal energy across all frequencies, pink noise has equal energy per octave, meaning the higher frequencies have less energy. This makes it a powerful tool in situations with background noise, like natural biological systems, where it mirrors the inherent noise.
Pink noise helps pinpoint subtle signals that would otherwise be masked by background noise. This is achieved by amplifying the effect of stochastic resonance—the phenomenon of weak signals being more easily detected in the presence of noise. Engineers can combine pink noise with a faint, repeating signal to reveal faint signals that would otherwise remain hidden. The application of pink noise reaches beyond audio, finding use in detecting signals in visual systems as well. Despite its potential, pink noise techniques have not been fully explored and present exciting opportunities for future audio engineers and researchers.
Pink noise is often overlooked as a tool for audio engineers, but it's surprisingly effective in revealing the acoustic properties of a room. Unlike white noise, which has equal energy at all frequencies, pink noise has equal energy per octave. This means its energy decreases with frequency, mimicking the way we actually hear sound.
The broadband nature of pink noise allows engineers to assess a room's acoustics across a broad range of frequencies, as opposed to focusing on individual frequencies. This comprehensive view is valuable for identifying problematic room modes - those frequencies where sound energy gets trapped, leading to uneven sound.
Because its energy is evenly distributed across the spectrum, pink noise is great for testing how systems handle natural sounds.
Using pink noise for calibration is standard practice in professional environments. By setting the signal level around 85 dB SPL, engineers can effectively evaluate how different audio systems respond to varying sound intensity levels.
While most focus on how pink noise affects amplitude, it's important to remember it also impacts the phase response of audio systems. By using pink noise, we can understand how a sound system affects the timing of different frequencies, leading to clearer sound and improved imaging.
It's often assumed that only complex techniques are useful in audio engineering. But as pink noise shows us, the simplest approaches can reveal surprising insights.
Mastering the Art of Resonance Detection 7 Practical Techniques for Audio Engineers - Modal Analysis Software for Complex Spaces
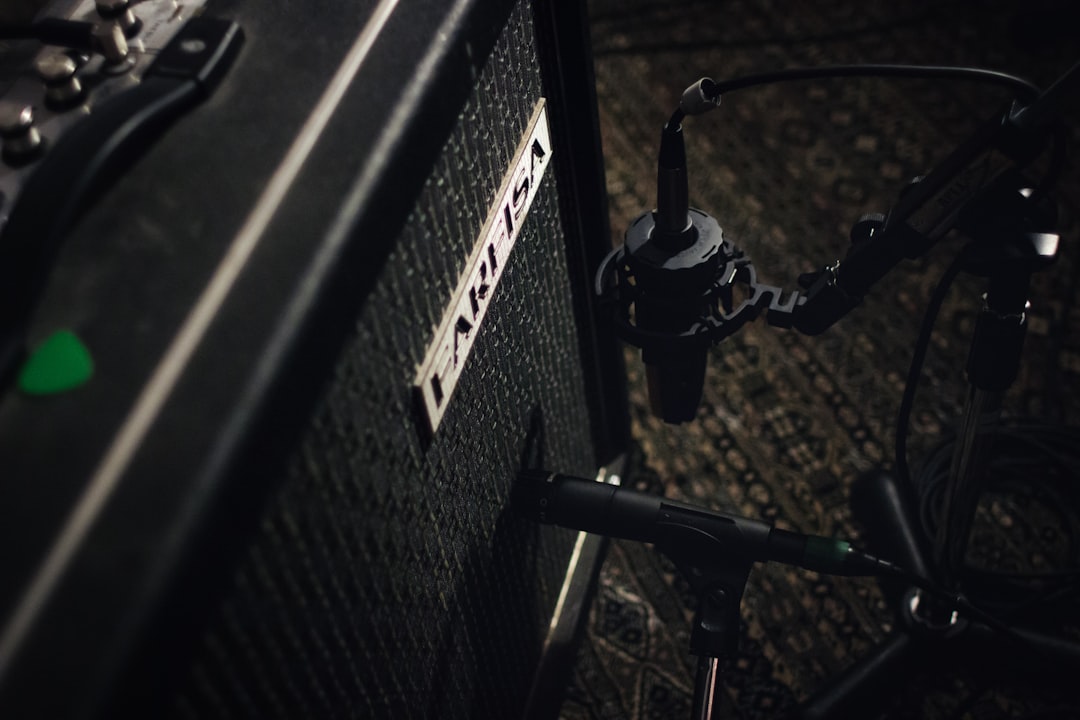
Modal analysis software is a valuable tool for audio engineers who are trying to master the art of resonance detection. It provides an in-depth look at the dynamic properties of a structure, such as natural frequencies and mode shapes. These insights are essential for troubleshooting issues like resonance frequencies and damping factors, which can directly impact the sound quality of a space.
Experimental modal analysis allows engineers to analyze a structure's behavior by exciting it with an impact hammer or modal shaker and then measuring the resulting vibrations. This software also enables the creation of geometries and the use of advanced analysis tools for extracting modal parameters from frequency response functions. This level of detail allows engineers to pinpoint problematic frequencies and design acoustic solutions for complex spaces.
Nonlinear modal analysis techniques, which can be applied to study nonconservative nonlinear systems, are becoming increasingly common in modern acoustic assessments. These sophisticated tools expand the capabilities of modal analysis software to include the study of dissipative systems and limit-cycle oscillations, leading to even more comprehensive and nuanced acoustic evaluations.
The advancements in modal analysis software are crucial for audio engineering. It helps engineers design and improve spaces, ensuring operational reliability and optimal sound quality for music recording studios, concert halls, and more.
Modal analysis software, which goes beyond just identifying resonant frequencies, can also reveal the intricate shapes and patterns of those resonances, called "mode shapes." This information is vital for engineers because it allows them to understand the complex ways that sound energy moves and spreads within complex structures. This knowledge can be used to optimize the design of these structures, as well as to develop targeted strategies for minimizing unwanted resonant effects.
Taking it a step further, modal analysis software can analyze how these resonant modes interact with each other in complex and irregularly shaped spaces like concert halls or studios. This is especially helpful in designing spaces that maximize the quality of sound, minimize unwanted echoes, and create the best possible listening experience.
The math behind modal analysis involves something called eigenvalue problems. It's a bit complex, but basically, the eigenvalues (numbers that come out of the math) represent the natural frequencies of a structure, while the eigenvectors (sort of like direction indicators) represent the corresponding mode shapes. This mathematical framework allows engineers to predict how a structure will resonate even before it is built, which can save a lot of time and resources.
Interestingly, some modal analysis software even uses stochastic techniques to analyze sound in real-world situations where there’s a lot of background noise, like at concerts or live events. This capability is really useful for engineers who are working in noisy environments where a perfectly controlled test is impossible.
The world of audio engineering is moving towards real-time analysis, and advanced modal analysis software is becoming increasingly capable of analyzing sound data in real-time. This capability is extremely helpful for live sound engineers who need to make adjustments on the fly, quickly identifying problematic frequencies and rectifying them to optimize the sound quality of a performance.
A recent development in modal analysis is the incorporation of machine learning. These tools can be trained on large datasets of previous analyses to predict potential resonance issues before they arise. This proactive approach can significantly improve the design process by identifying problems early on.
There’s an additional aspect of modal analysis that’s useful for multi-component systems like musical instruments or large arrays of speakers. This software can analyze how the different parts of the system interact with each other to influence the overall resonant behavior. This insight helps engineers to fine-tune performance and ensure that the system behaves as intended.
Traditional modal analysis tends to rely on linear models, but new software tools are moving into the world of non-linear analysis. This allows engineers to understand how their systems might behave differently under different operating conditions, enhancing the resilience of their designs.
To help engineers visualize the complex interactions between different modes, some modal analysis software offers 3D visualizations. These visualizations allow engineers to actually “see” how sound reflects, bounces, and interacts with various surfaces in a space, making it easier to understand and solve complex acoustic problems.
Finally, modal analysis can go beyond just identifying problems. Some software allows engineers to perform sensitivity analysis, which helps them understand how the system's resonant characteristics might be affected by changes to the structure's shape, its mass distribution, or the way it interacts with its surroundings. This tool helps engineers to fine-tune their designs before committing to a final product.
More Posts from transcribethis.io: